The concept of traveling waves is basic to understanding what's happening in antennas and transmission lines. We take these things for granted in most everyday life experiences since the wavelengths involved are such that t-line (i.e. transmission line) effects are either unnoticeable or are so small that they can be ignored. But the concepts hold even when we ignore them.
When the length of a transmission line gets to about 1/4 of the wavelength then t-line effects become quite pronounced and cause serious system degradation if they are not accommodated in the design. For RF systems, say your WiFi router running at 2.4 GHz, the wavelength is a mere 12.5 cm. So any transmission line (i.e. wire carrying that signal) that is longer than 3.5 cm (i.e. 1/4 of the wavelength) must be designed with things like impedance matching in mind.
Ok, so what is "characteristic impedance" or Z0? If you recall Ohm's Law you know that it says:
R = V/I
So this same concept can be applied to a transmission line or an antenna which is nothing but a device to couple electrical signals in a wire to electromagnetic waves in (on earth at least) the air. The value of Z0 is nothing but the ratio of the voltage and the current of the signal in the transmission line. For commonly used 50 Ohm coax cable the ratio is 50, hence 50 Ohm cable.
All is well if we have a very long line, perhaps infinitely long in theory. We put a signal in at one end and away it goes. We never hear about it again at the driven end. But there is no such cable in the world we live in. Cables have finite length and let's say there is a 100 m cable with a cut end. So we now have a discontinuity there.
It's important to understand what happens when our wave, travelling along happily in a 50 Ohm cable does when it hits this infinite Ohm endpoint. What happens is the signal is reflected back to the source. In the case of an open-circuit we get 100% reflection and, of course, the original signal and the reflected signal are summed together in the wire. The net effect is that if we are sending 1 V down the line, we end up with 2 V when the original and reflected wave are added together.
If the endpoint is not a short circuit but rather 25 Ohms, we then don't get 100% reflection but only 33% because we use the formula for calculating reflection:
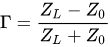
Nothing to complicated about this equation, it's just the ratio of the difference of the load and line impedance and the sum of the load and line impedance. (If you choose to study electrical engineering at a university, you will learn this and how to derive it but at this point it's simple enough to use.)
In any case, however, reflected waves are undesirable because if we are driving an antenna, for example, any power reflected is power that did not go into the antenna and is reflected back to our transmitter. Not only does that reduce the output of the antenna it also may damage the transmitter if the ratio of the reflected signal to the driven signal is too high. So we want to match the impedance of the output of the transmitter, the transmission line, and input to the antenna, and the output of the antenna so that as close to 100% of the power we get out of the transmitter ends up as radiated RF in the air.
So how do we do that? The transmitter itself is most often designed to work with standard transmission lines like 50 Ohm. So using 50 Ohm coax (or whatever the transmitter is designed for) will give us close to 100% coupling from the transmitter to the line. Next is the antenna. Most commercially made antennas have an input impedance of 50 Ohms since that type of coax is common but the antenna itself may have a balun (a transformer that couples single-ended coax to a double-ended antenna like a Yagi design) or not if the antenna is a single ended design like the one on your WiFi router. But the important thing is that the antenna is designed or tuned to look like a 50 Ohm load to the coax.
The next thing is the antenna itself and this is as much art as it is science. The first problem is that air or free space itself has a characteristic impedance just like the coax and that number is about 377 Ohms. If we don't handle this in our antenna design we will have a large impedance mismatch between our 50 Ohm coax input to the antenna and the 377 Ohm impedance of the air we're trying to drive a signal into. The formula above gives a factor of 0.76 for this case which means for every Watt we put into the antenna, only 0.76 Watts gets transmitted. The other 24% gets reflected back and does no good. Now you could operate like this but it can, as mentioned earlier, cause damage or cause instability in the circuits of the transmitter.
The job of an antenna designer is to get as close to 100% coupling of the signal input to the antenna into the air. There are various ways of doing this but I'd encourage you to start your research using some of the materials that are available to Amateur Radio operators in your country. In the USA there is an organization called the ARRL that publishes a lot of good technical information on how to design antennas and I suspect your nation (if you are not in the USA) has a similar organization. If not, I am sure you can get ARRL materials over the internet.
You might start here:
ARRL Antenna Modelling
The EZNEC software is free and very good for antenna design and modelling and will likely help you get started:
EZNEC Software
I hope this helps you get started. The theory behind all this can be somewhat intimidating but in practice it can be done with a bit of study and experimentation.